Surgeons are fascinated by the disease processes that lead to conditions which require surgical intervention. Because they intervene directly in these disease processes at later stages, surgeons gain a unique vantage point with which to propose the optimal course of treatment for disease at earlier stages. In the following series of blog posts, we’ll be looking at the nanoparticle research efforts of Dr. Ian McGilvray to understand the inner workings of the liver and how liver cancer might be thwarted with the use of cutting edge nanotechnology.
In 2003 Dr. Ian McGilvray founded a research lab at Toronto General Hospital, initially looking at genomics associated with Hepatitis C (HCV) and Hepatocellular Carcinoma (HCC) therapies. Since then, the McGilvray lab has grown and published several papers on diseases and treatments related to the liver. In 2015, Dr. McGilvray established the Toronto Liver Laboratory with the aim of reprogramming the liver to treat hepatic disease. In the past few years, the team has published key papers centered around this topic. Together these papers are stitching together a story; first describing a baseline, then looking at therapeutic possibilities.
Assistant Scientist at the Toronto Research Institute, Dr. Sonya MacParland was recruited in 2017 to become a PI in the Toronto Liver Laboratory and to establish her independent group studying the immune microenvironment in the liver. A PhD in Immunology from Memorial University of Newfoundland, Dr. MacParland had been doing her post-doctoral studies at the University of Toronto under the supervision of Dr. Mario Ostrowski.
NANOPARTICLE RESEARCH
A significant focus of the research in the Toronto Liver Laboratory has been the potential to use nanoparticles for cancer treatment. Inorganic particles between 1 and 100 nanometres (nm) in size, nanoparticles have unique properties that give them distinct advantages and present interesting challenges for clinical application.
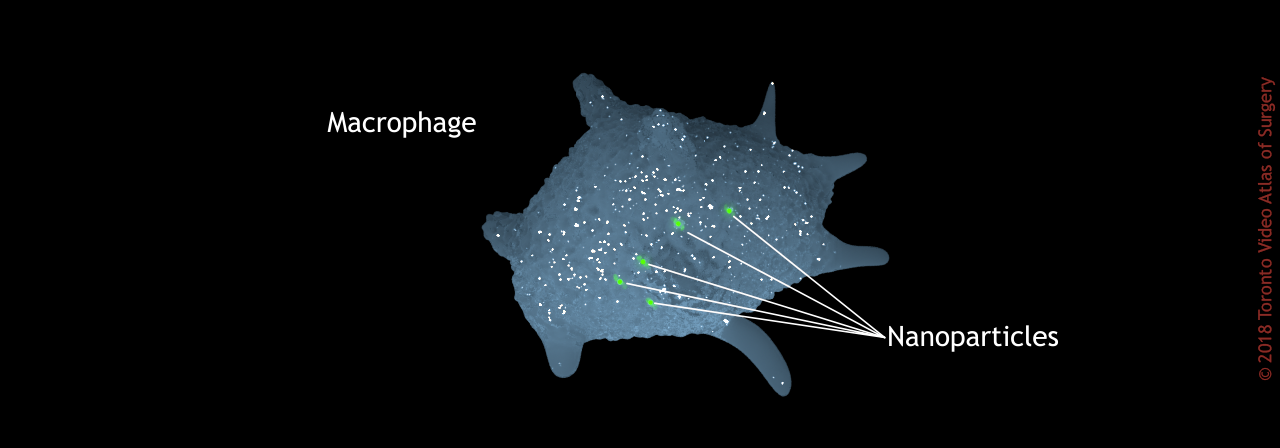
Relative size of nanoparticles to macrophage.
One advantage to using nanoparticles is that they can be delivered in a targeted way, and can then be tracked with proteins that are added to the nanoparticle to make them fluoresce. They can also be loaded with a payload such as a chemotherapeutic. When patients undergo chemotherapy, that chemotherapy goes systematically throughout the whole body, and unfortunately there can be many off-target effects. Alternatively, by loading nanoparticles with chemotherapeutics, and injecting to a targeted location, the chemotherapeutic could go directly to the tumour, and off-target, systemic, cytotoxic side-effects could be avoided. Another aspect to consider when using nanoparticles to attack cancer, is that tumours tend to have “leaky” vasculature (destructed epithelium), so the nanoparticles can go in quite easily.
The biggest challenge is that the majority of nanoparticles, no matter what you target them with, and where you intend them to go, will be cleared from the bloodstream by a network of immune cells that are found in the liver, spleen and bone marrow. As much as 99% will be taken up by the liver, in what is called a first-pass effect. The next major biological barrier that captures and “sequesters” nanoparticles aside from the liver, is the spleen.
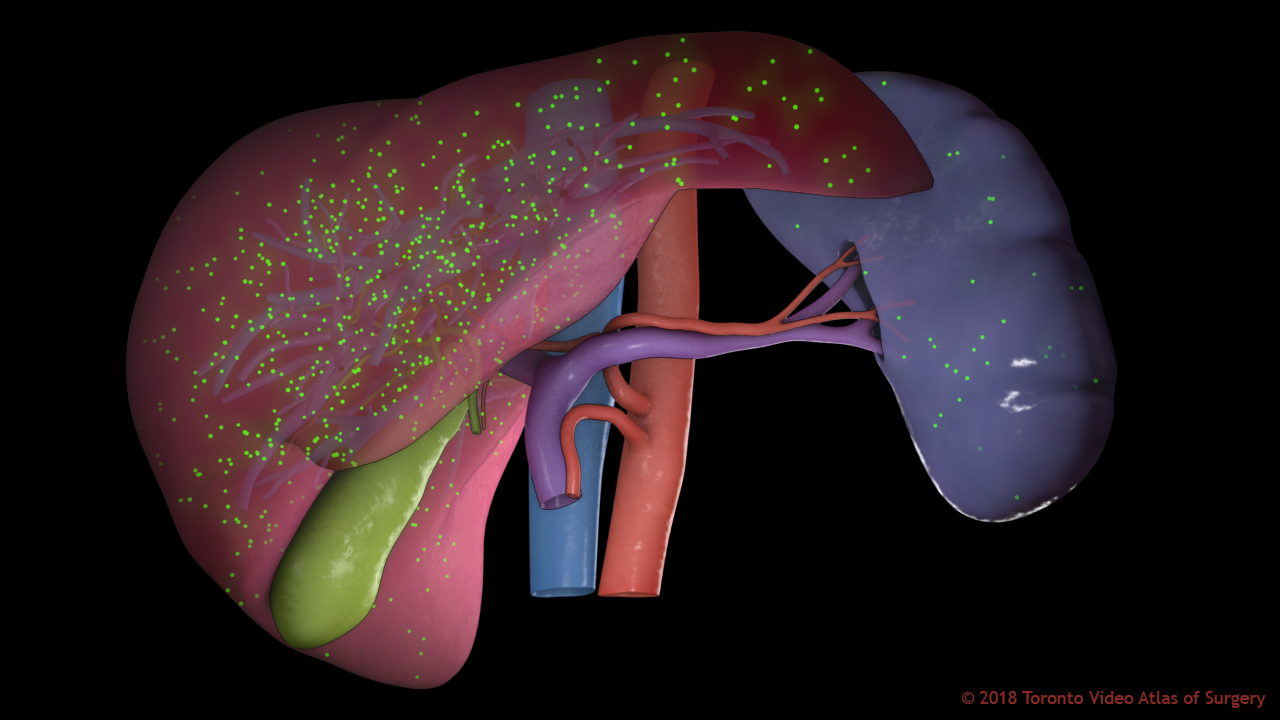
Relative distributions of nanoparticles sequestered in liver & spleen (nanoparticles not to scale).
Macrophages are immune cells that eat invasive particles, and are found throughout the body. There are a population of macrophages which are specialized to perform only within a specific organ or tissue of the body, called “tissue-resident macrophages”. The majority of tissue-resident macrophages are found in the liver.
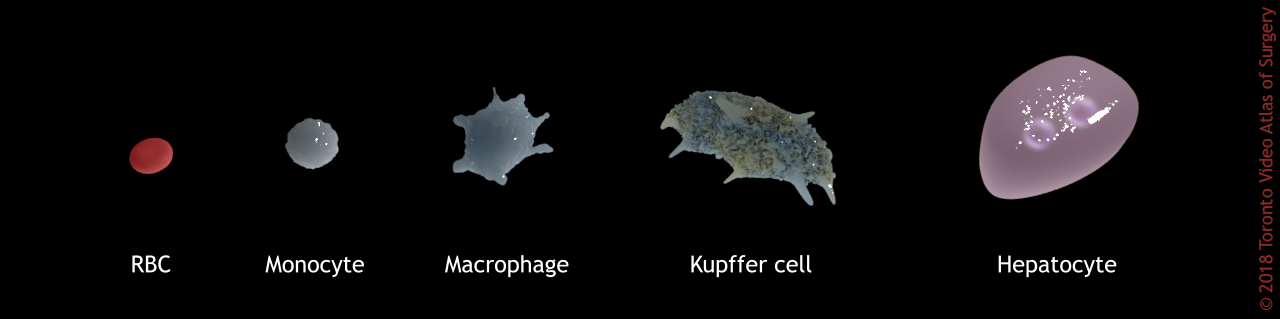
Relative size of cells: RBC (red blood cell), monocyte (immune cells that circulate in the blood), macrophage (immune cells that eat invasive particles), Kupffer cell (liver-specific macrophage), hepatocyte (functional liver cell).
Liver macrophages, aka “Kupffer cells” appear to be the culprits when it comes to sequestering nanoparticles. They are meant to clear bacteria from the gut, play a homeostatic role, an immunoregulatory role, and pro-inflammatory role in the liver. Interestingly, macrophages in the spleen, which serves similar physiological roles to the liver, do not take up as many nanoparticles.
DELIVERING NANOPARTICLES
A major question this research aimed to address was to the problem of nanoparticles getting trapped in the liver. They wanted to find out what exactly in the liver was absorbing nanoparticles and suggest strategies for future research. Possible explanations for sequestering of nanoparticles in the liver was blood flow dynamics, organ microarchitecture, or cellular phenotype (a cell's particular form and function, or specialization). In 2016 the team published a paper in Nature Materials that demonstrated their findings:
Abstract
The liver and spleen are major biological barriers to translating nanomedicines because they sequester the majority of administered nanomaterials and prevent delivery to diseased tissue. Here we examined the blood clearance mechanism of administered hard nanomaterials in relation to blood flow dynamics, organ microarchitecture and cellular phenotype. We found that nanomaterial velocity reduces 1,000-fold as they enter and traverse the liver, leading to 7.5 times more nanomaterial interaction with hepatic cells relative to peripheral cells. In the liver, Kupffer cells (84.8 ± 6.4%), hepatic B cells (81.5 ± 9.3%) and liver sinusoidal endothelial cells (64.6 ± 13.7%) interacted with administered PEGylated quantum dots, but splenic macrophages took up less material (25.4 ± 10.1%) due to differences in phenotype. The uptake patterns were similar for two other nanomaterial types and five different surface chemistries. Potential new strategies to overcome off-target nanomaterial accumulation may involve manipulating intra-organ flow dynamics and modulating the cellular phenotype to alter hepatic cell interactions.
When blood enters the liver, it travels through a labyrinth of vessels called sinusoids. Blood flow slows down in the liver sinusoids, allowing for increased interactions between particles floating in the blood with both circulating and stationary cells. The removal of nanomaterials from the blood was carefully analyzed using a mathematical model that took blood vessel length, diameter and blood flow velocity into consideration.
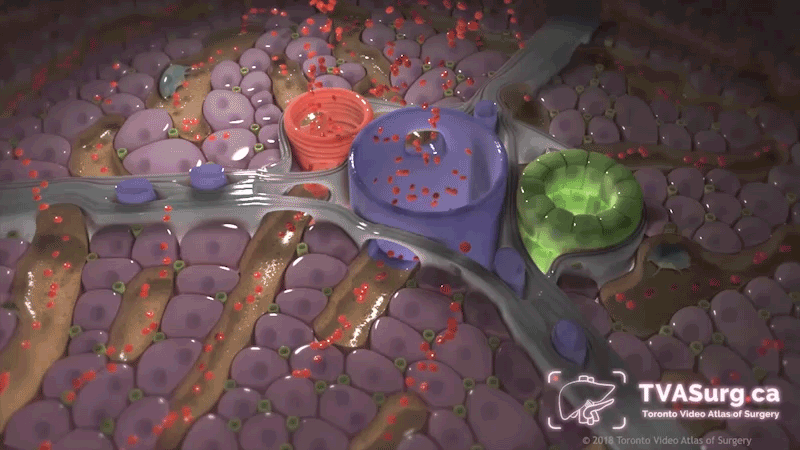
Blood flow slows down after entering liver lobules.
The study found that nanomaterial velocity in the blood was reduced 1,000-fold when traversing the liver, leading up to 7.5 times more interactions with liver cells as opposed to cells outside the liver. The importance of flow dynamics is reinforced by the fact that, under static culture conditions, monocytes (immune cells that circulate in the blood) took up nanoparticles with the same affinity as Kupffer cells.
When looking at the microarchitecture of the liver, the study focused on the inflow of blood through portal triad zones, where blood enters the liver lobules, and compared what was happening there to what was happening at the central vein regions, where blood flow exits the neighbourhoods of hepatic liver cells and begins its departure out of the liver.
There was a higher amount of nanoparticle accumulation in the zone surrounding the portal triad than in the zone surrounding the central vein. So a cell located near the vascular inlet is more likely to take up a nanomaterial, and this cell will accumulate more nanomaterial when compared to a cell located near the vascular outlet.
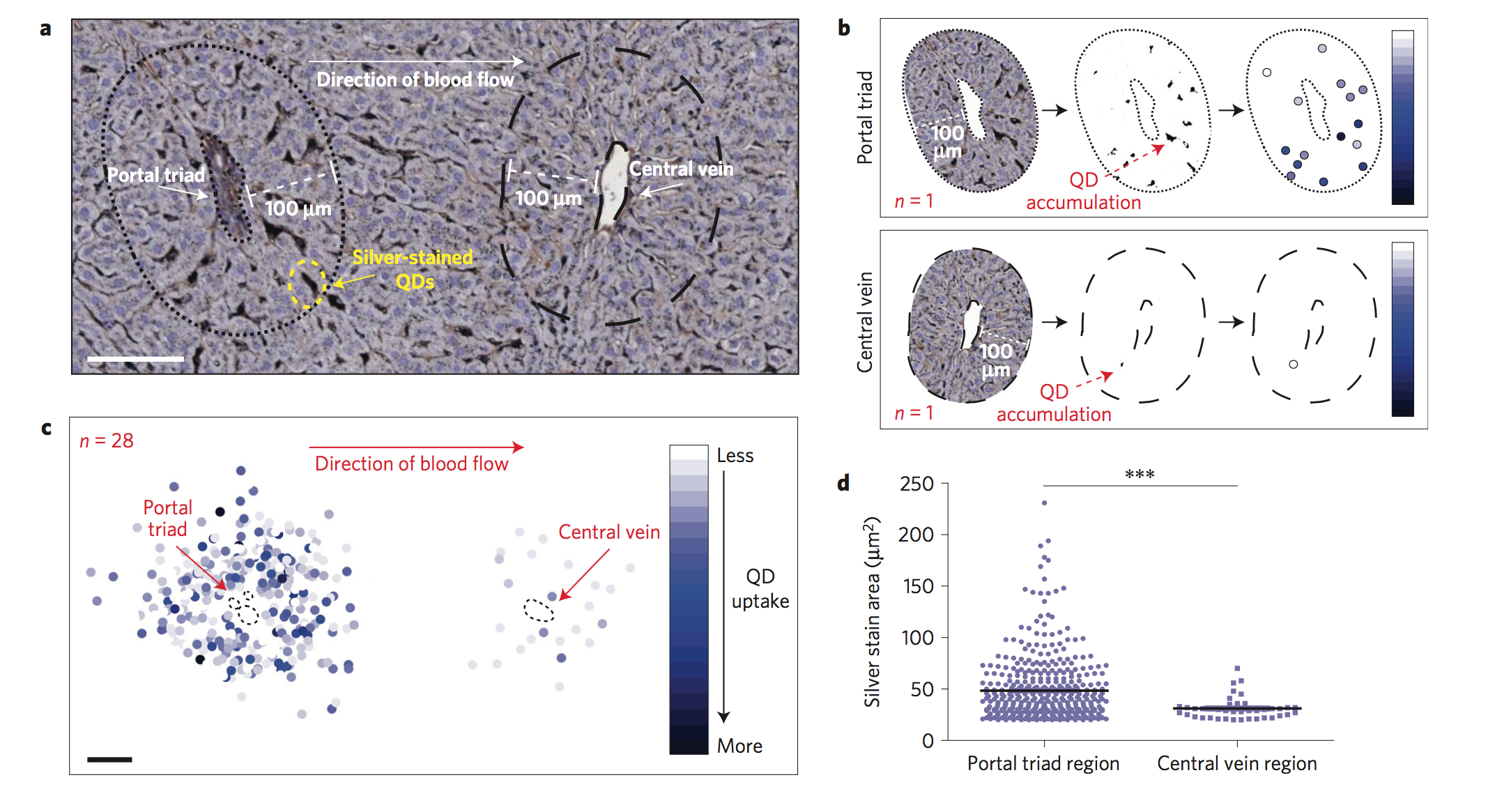
Results of the study showed that nanoparticles were being captured rapidly upon entering the liver.
MACROPHAGE PHENOTYPE
There are different types of macrophages in the body, and each has several subtypes. The cellular phenotype of macrophages was investigated to see what effect that has on the cell’s ability and affinity for taking up nanoparticles. The surface expression of various receptors, and what kind of cytokines (communication proteins secreted by immune cells) were being released was analyzed to determine phenotype.By comparing macrophages from the spleen to macrophages from the liver, the team noticed a difference in their inclination for taking up nanoparticles. When they analysed nanoparticle uptake in splenic macrophages, they found that these cells took up significantly less nanoparticles than liver Kupffer cells. In fact, splenic macrophages took up ten times less nanomaterial on a per cell basis. So liver macrophages specifically are avidly consuming nanoparticles, compared to immune cells from other parts of the body.
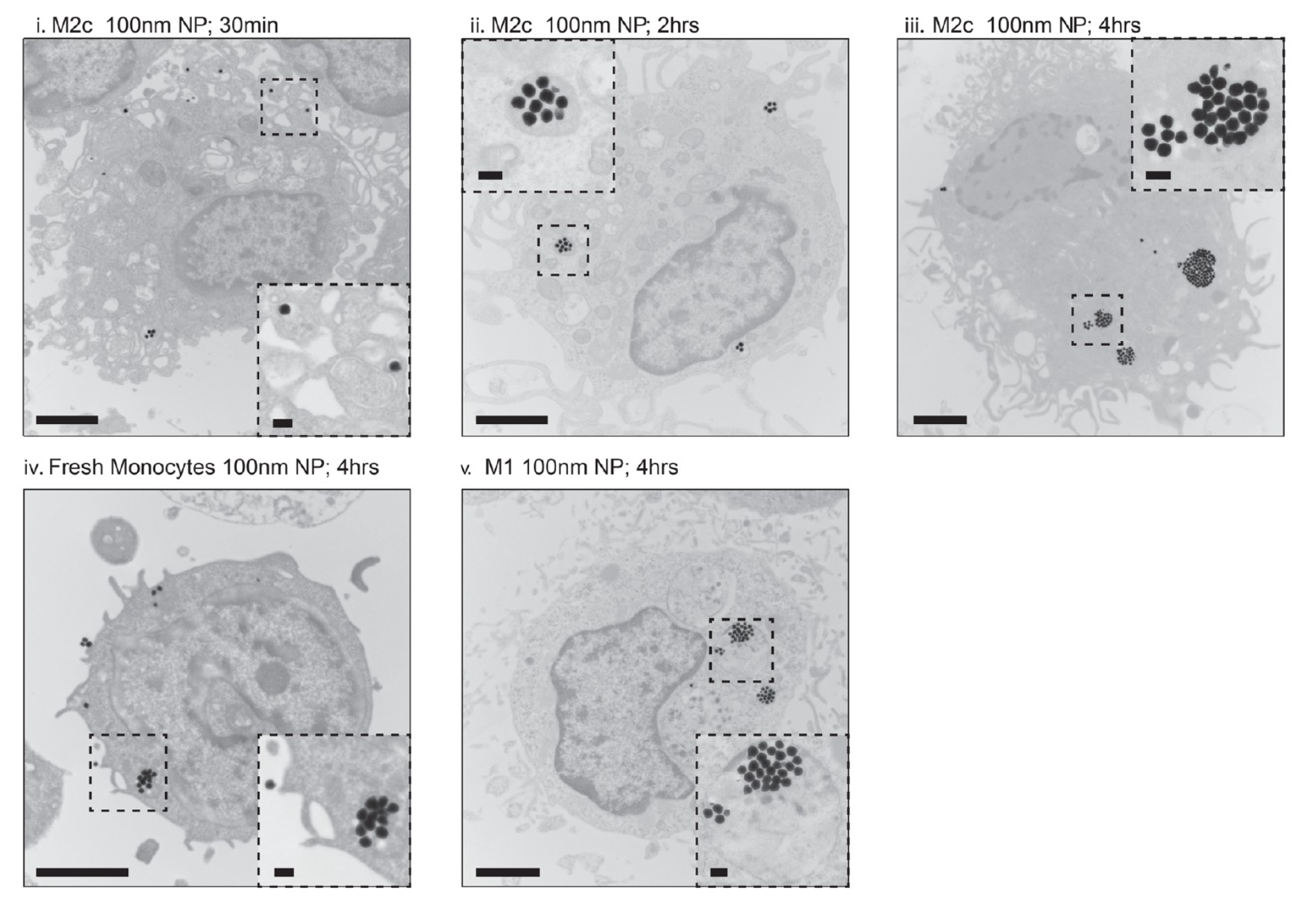
Histologic image of nanoparticles concentrated in liver macrophages.
Macrophages are products of their environment. Liver macrophages may be produced natively within the liver, or be recruited from the periphery, in which case, when they arrive there must be something in the tissue that assigns them their role. This will usually be locally released cytokines or neighboring cells. Inflammation is a major reason why local cells will release cytokines.
In general, liver macrophages are immunoregulatory by default (the M2 type), meaning they express receptors that are really good at picking up stuff. Their job is to survey, clean and repair, and they secrete cytokines that tell neighboring immune cells in the liver that “all’s clear.” It is known that if a macrophage is placed into a more inflammatory environment, its surface will change; they will down-regulate cleaning and repairing surface receptors, and release different cytokines to recruit other inflammatory immune cells (the M1 type).
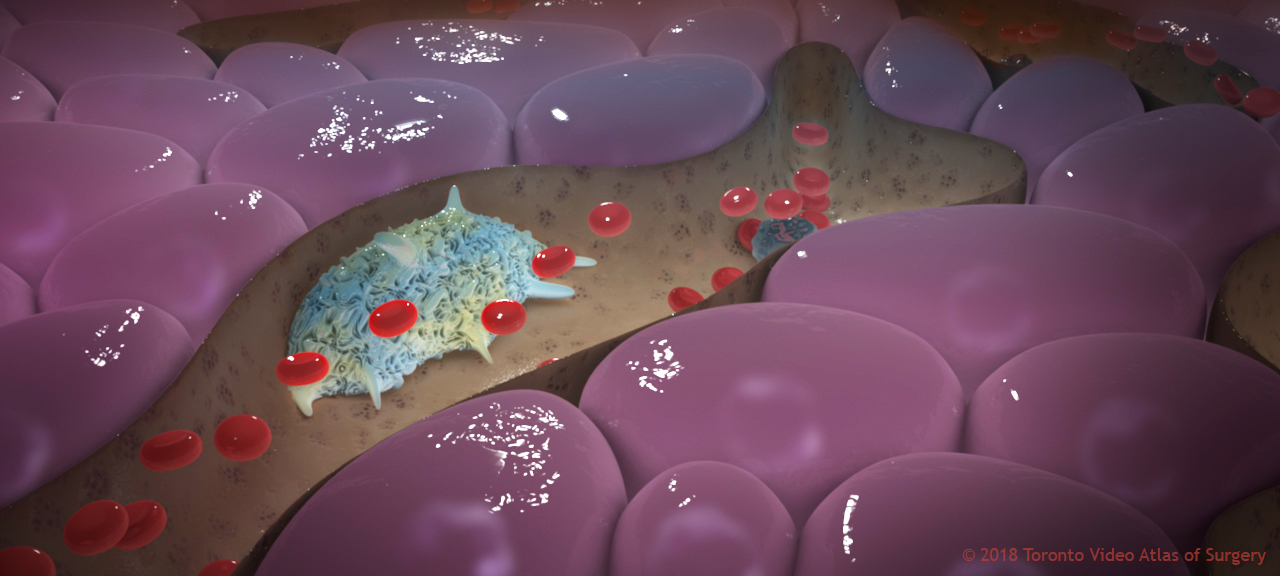
Liver macrophages are immunoregulatory by default (M2). They express cell surface scavenger receptors and produce high amounts of anti-inflammatory cell signalling proteins, dampening the immune response.
In vitro, cytokines were introduced that would mimic inflammation of the liver, leading macrophages to change their surface phenotype. The study found that by changing the liver macrophage phenotype, it changed the amount of nanoparticles that are taken up. In this case, by changing the macrophages to the M1 inflammatory phenotype, the nanoparticles were taken up 40% less.
Nanoparticle material type and design was also taken into consideration. Various sizes of nanoparticles were investigated, as well as the use of a specialized coating to prevent clumping. Even when the optimal sized nanoparticles were coated with an agent to prevent absorption, significant absorption in the liver was maintained, demonstrating that modifications to the nanoparticle size and surface properties will not single-handedly solve the delivery problem. The study instead suggests that manipulation of the biological environment should be pursued.
The findings of this multi-part study indicated potential new strategies for nanomedicine to circumvent the problems of liver sequestration. Future applications of nanoparticles in humans might have to consider altering blood flow dynamics and/or manipulating immune cell phenotype. By testing uptake in Kupffer cells with static, slow and fast flow rates, increasing the flow rate reduced nanoparticle uptake.
Thanks for reading about the nanoparticle research that has been conducted at the McGilvray & MacParland Labs! If you'd like to learn more, you can reach Dr. Ian McGilvray at Ian.McGilvray@uhn.ca and Dr. Sonya MacParland at Sonya.MacParland@uhnresearch.ca
Stay tuned for our next post, where we'll take a closer look at how different immune cell populations interact with nanoparticles, and discuss future directions of research in this niche area.